Sterilization
1Q2024 Healthcare Recalls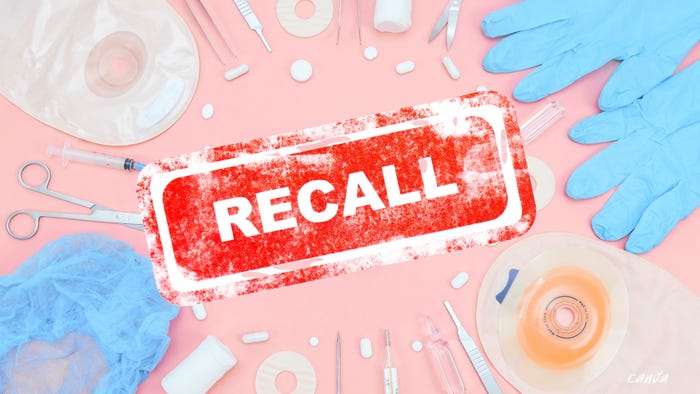
Packaging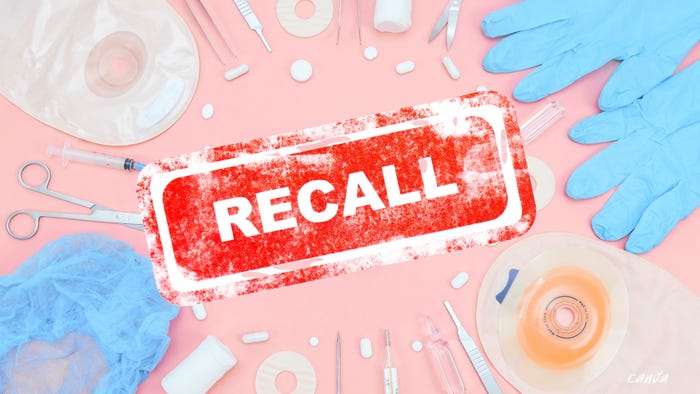
Disturbing Healthcare Recalls in 1Q2024Disturbing Healthcare Recalls in 1Q2024
Take a closer look at some of FDA’s recent pharmaceutical and medical device recalls where packaging has played a role.
Sign up for the QMED & MD+DI Daily newsletter.